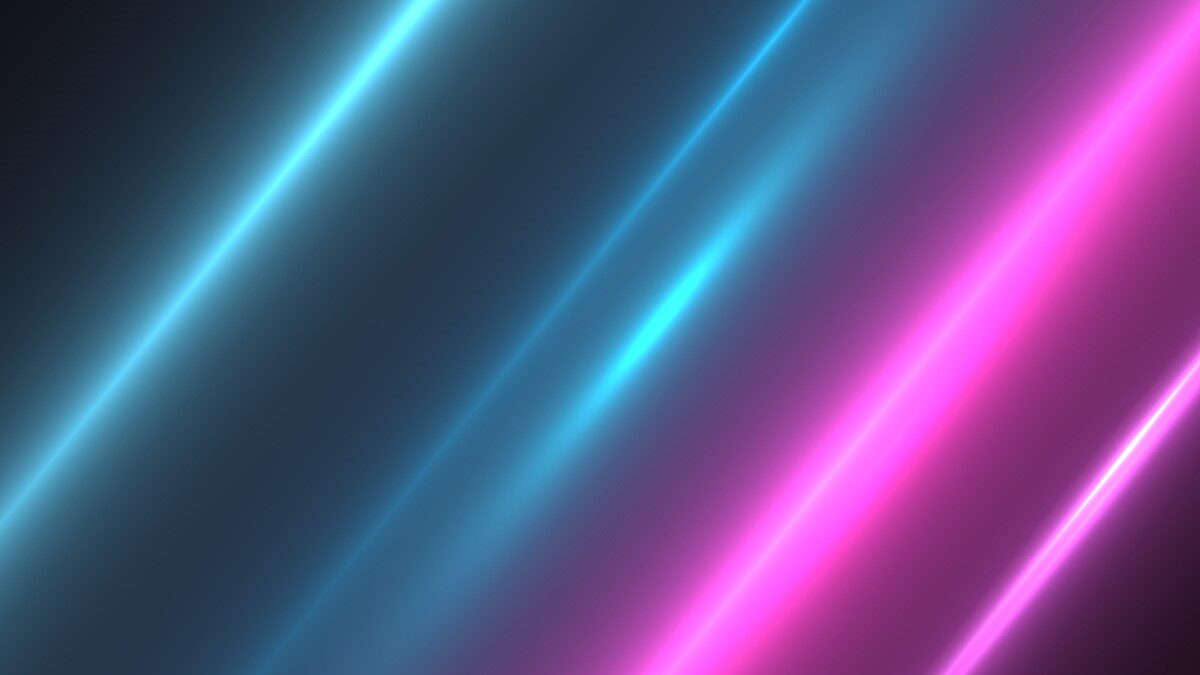
Aephraim Steinberg and colleagues at the University of Toronto made the discovery while investigating what happens to a beam of photons passing through a cloud of atoms when the photons' frequency is equal to that of one of the atomic transitions. Intuitively, they say, it would be expected that those photons exciting atoms within the cloud would be absorbed and then at best re-emitted in a random direction. As such, the flux of photons coming from excited atoms that are detected in the forward direction would be miniscule.
Indeed, they point out, this idea that only absorbed, or "lost", photons contribute to the excitation springs naturally from theory that tells us the total time atoms spend in the excited state is directly proportional to the number of photons that are lost.
Atomic cloud
To explore this idea further, Steinberg and colleagues have now tied atomic excitations to the detection of individual photons arriving at a detector on the far side of an atomic cloud - to establish whether those photons really contribute nothing to the excitations. They did so not with single photons as such, but instead used single-photon detection to establish when an interacting light pulse's mean number of photons increases by one.
Their experiment consisted of an ultracold cloud of rubidium-85 atoms stored in a magneto-optical trap and exposed to two counter-propagating laser beams. One of these beams, called the "signal", was pulsed and resonant with one of rubidium's transitions, and ended up at a photon detector on the far side of the cloud. The other beam, called the "probe", was set off-resonance and used to record the phase change associated with atomic excitation. This is possible because the atoms' ground and excited states having different refractive indices.
The idea was to measure the degree of phase change both with and without a detector click, placing the two sets of results in separate bins. In this way, the researchers could establish for how long the lost and transmitted photons excited the atoms for.
Phase shift
The researchers used signal pulses lasting 10 ns each and emitted a little less than twice a microsecond, with some 60% of the shots being absorbed by the optically-thick medium. By measuring the phase once every 16 ns they were able to distinguish the single pulses' phase shift from other variations in the medium's refractive index. They also screened out other sources of noise that could have generated spurious correlations between the phase of the probe beam and the single-photon detector.
As they report in a paper accepted for publication in PRX Quantum and uploaded to the arXiv preprint server, they gathered data for over 100 h and recorded about 6 billion signal pulses. They say they were clearly able to identify the signal pulses' modulation of the probe's phase and use their data to compare the phase shift with and without a detector click. Their conclusion is that the phase shift caused by a transmitted photon amounted to 77% of the shift due to an average incident photon.
"The unequivocal implication of this result is that even photons which are ultimately transmitted spend a significant portion of time as atomic excitations," they write.
Trying to explain their results physically, the researchers say that transmitted photons could naively be expected to excite atoms over longer periods of time than could lost photons - owing to their ability to polarize atoms over the entire length of the sample. But they say that this "egalitarian" model generates physically implausible results for very thin samples.
Photons "switch teams"
They argue instead that "coherent forward emission" - which arises naturally when broadband pulses propagate through an optically thick medium with frequency-dependent absorption - is likely responsible. As Steinberg explains, the phase of a photon's electric field determines whether the field excites an atom or drives it back down to its ground state. Because broadband pulses develop phase flips, they can start by exciting an atom but then "switch teams" and send the atom back to its ground state before it naturally decays. And when this happens, the released energy is not emitted randomly but instead in the forward direction.
To try and develop a full quantum-mechanical understanding of their latest result, Steinberg and colleagues have teamed up with quantum theorist Howard Wiseman of Griffith University in Australia. They say they have "made a lot of progress" in this direction and are also working on a new round of experiments to test some of the theory's predictions. This work, they add, will involve varying the atomic cloud's optical depth as well as the laser bandwidth and pulse shape, in order, as they put it, "to further elucidate the strange history of transmitted photons".
Commenting on the work, Howard Carmichael of the University of Auckland in New Zealand says that he is not surprised by the result. He explains that an optically thick medium behaving linearly, in which the atoms are close to their ground state, would be expected to absorb incoming resonant photons. In contrast, he argues, a nonlinear medium with most atoms in the excited state would instead be expected to transmit the light. There is, he says, "an implied correlation" between the transmission and the absorber being excited rather than in its ground state.
Steinberg, however, takes issue with this interpretation. "Our absorbers stay close to the ground state yet absorb about half of the incident photons," he says. "The fact that on occasion we find a transmitted photon certainly does not prove that the absorber was in the excited state."
Everything that is, is made of Light.
Light is a Field, not scattered particles.