Glial cells, which help neurons communicate with each other, can leave the central nervous system and cross into the peripheral nervous system to compensate for missing cells, according to new research in the Dec. 2 issue of The Journal of Neuroscience. The animal study contributes to researchers' basic understanding of how the two nervous systems develop and are maintained, which is essential for the effective treatment of diseases such as multiple sclerosis.
The nervous system is divided into the central nervous system (the brain and spinal cord) and the peripheral nervous system (sensory organs, muscles, and glands). A major difference between the systems is that each has its own type of glial cells. In a healthy body, glial cells are tightly segregated and aren't known to travel between the two systems. The peripheral nervous system also regenerates more than the central nervous system, due in part to its glial cells -- a characteristic that, if better understood, might be used to improve the regenerative capabilities of the central nervous system.
Glial cells serve nerve cells by insulating them with layers of fats and proteins called myelin. Myelin coatings are necessary for nerve signals to be transmitted normally; when the sheaths are lost, disorders involving impairment in sensation, movement and cognition such as multiple sclerosis or amyotrophic lateral sclerosis develop. Glial cells named oligodendrocytes produce myelin around nerves of the central nervous system, while those named Schwann cells make myelin that insulates peripheral nerves.
Some of the common words we use are frozen mistakes. The term influenza comes from the Italian word meaning "influence" - an allusion to the influence the stars were once believed to have on our health. European explorers searching for an alternate route to India ended up in the New World and uncomprehendingly dubbed its inhabitants indios, or Indians.
Neuroscientists have a frozen mistake of their own, and it is a spectacular blunder. In the mid-1800s researchers discovered cells in the brain that are not like neurons (the presumed active players of the brain) and called them glia, the Greek word for "glue." Even though the brain contains about a trillion glia - 10 times as many as there are neurons - the assumption was that those cells were nothing more than a passive support system. Today we know the name could not be more wrong.
Glia, in fact, are busy multi-taskers, guiding the brain's development and sustaining it throughout our lives. Glia also listen carefully to their neighbors, and they speak in a chemical language of their own. Scientists do not yet understand that language, but experiments suggest that it is part of the neurological conversation that takes place as we learn and form new memories.
Microglia, immune cells in the brain, are suggested to be involved in the repair of damaged brain, like a medical doctor. However, it is completely unknown how microglia diagnose damaged circuits in an in vivo brain.
Now, a Japanese group led by Professor Junichi Nabekura and Dr Hiroaki Wake of National Institute for Physiological Sciences, NIPS, Japan, has successfully taken a live image how microglia survey the synapses in the intact and ischemic brains of mice by using two-photon microscopic technology.
They report their finding in Journal of Neuroscience on April 1, 2009.
In their study, the researchers took an intense tune-up of their two-photon microscopy and achieved to visualize the fine structures of neurons and glia of mice in the range of 0 to 1 mm from the brain surface (world-leading deep imaging technology).
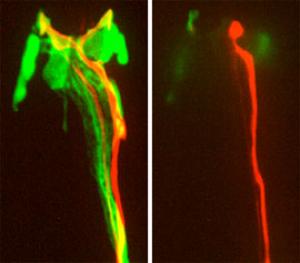
© Rockefeller UniversityFrayed nerves. New research in a C. elegans sensory organ shows that the branch-like ends of neurons (red) shrivel into nubs in the absence of glia (green).
Sensory neurons have always put on a good show. But now, it turns out, they'll be sharing the credit. Rockefeller University scientists now show that while neurons play the lead role in detecting sensory information, a second type of cell, the glial cell, pulls the strings behind the scenes.
The findings, point to a mechanism that may explain not only how glia are required for bringing sensory information into the brain but also how glia may influence connections between neurons deep within in it.
"This is a convincing demonstration that glia play an essential role in the function of the nervous system," says Shai Shaham, head of the Laboratory of Developmental Genetics. "Without sensory neurons, animals can't sense their environment and react to it. What we found is that glia are required for the activity of these neurons and that glia are required to establish the quality of the animal's response to its environment."
During this year's baseball playoffs, Chicago White Sox outfielder Ken Griffey Jr., 38, threw a picture-perfect strike from center field to home plate to stop an opposing player from scoring. The White Sox ultimately won the game by a single run and clinched the division title. Had Griffey been 40, it could be argued, he might not have made the throw in time. That's because in middle age, we begin to lose myelin - the fatty sheath of "insulation" that coats our nerve axons and allows for fast signaling bursts in our brains.
Reporting in the online version of the journal Neurobiology of Aging, Dr. George Bartzokis, professor of psychiatry at the UCLA Semel Institute for Neuroscience and Human Behavior at UCLA, and his colleagues compared how quickly a group of males ranging in age from 23 to 80 could perform a motor task and then correlated their performances to their brains' myelin integrity. The researchers found a striking correlation between the speed of the task and the integrity of myelination over the range of ages. Put another way, after middle age, we start to lose the battle to repair the myelin in our brain, and our motor and cognitive functions begin a long, slow downhill slide.
The myelination of brain circuits follows an inverted U-shaped trajectory, peaking in middle age. Bartzokis and others have long argued that brain aging may be primarily related to the process of myelin breakdown.
By mopping up excess neurotrophic factor from neuronal synapses, astrocytes may finely tune synaptic transmission to affect processes such as learning and memory, say Bergami et al.
The major cellular events of learning and memory are long-term potentiation (LTP) and long-term depression (LTD), both of which affect neurons' ability to communicate with one another. Neurons that have undergone LTP display a stronger electrical response to the same level of a stimulus, whereas neurons that have gone through LTD display a weaker response. These changes are thought to result from modifications of the neuronal synapses, such as alterations in the density of postsynaptic receptors, or downstream signaling events.
Secretion of the neurotrophic factor BDNF (brain-derived neurotrophic factor) has been implicated in long-term synaptic modification, and the function of BDNF on synaptic strength depends on its particular form: in its pro-BDNF form it is believed to promote LTD, and in its mature form it prompts LTP.
Researchers at Georgetown University Medical Center have discovered a new way to limit inflammation caused by the activation of microglia - key immune cells in the brain. Although the role of such cells is to "clean up damage" after injury, they often worsen the damage by releasing toxic inflammatory factors.
In the October issue of the journal Glia, now published online, the scientists say that the type of chemical they used to deactivate these cells could possibly be developed as a drug to treat a variety of acute and chronic disorders marked by brain cell damage - including stroke, head and spinal cord injury, and possibly Alzheimer's disease and Parkinson's disease.
"Inflammation associated with the activation of microglial cells is an important factor that appears to contribute to tissue damage and disability in many of the important neurodegenerative disorders. By decreasing this inflammatory response, tissue loss after injury can be reduced. Thus, what we found in this study has important potential therapeutic implications for the treatment of a number of important neurological disorders," says the study's senior investigator, Alan I. Faden, M.D., a professor of neuroscience and director of the Laboratory for the Study of Central Nervous System Injury.
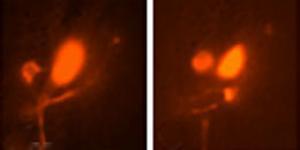
© Rockefeller UniversityMind-blowing. In C. elegans, fluorescent images show that when brain cells called glia are zapped -- and killed -- with a microscopic laser, neurons (red) survive but are highly abnormal (right) compared to normal ones (left).
Again and again, experiments confirmed it. Without glia, neurons die. So scientists who wanted to study in living animals what glia - the most abundant brain cells - do for neurons besides keep them alive were out of luck. But now, a breakthrough.
A system unveiled and described by Rockefeller University scientists shows that in the
Caenorhabditis elegans worm, neurons live on despite the absence of glia, a landmark discovery that paves the way for scientists to explore the dialogue between these team players in their natural environment.
"As far as we know, this is the first system where removing glia does not affect neuronal survival," says Shai Shaham, head of the Laboratory of Developmental Genetics, who made the discovery along with graduate student Satoshi Yoshimura. "So now we can study glia and the contributions they make in the developing brain in this
in vivo context."
To allow nerve cells to transmit information efficiently over long distances, advanced life forms have developed a mechanism known as saltatory conduction. This is made possible by an insulating sheath of myelin that forms at certain intervals around the axonal extensions of nerve cells that specialise in the transmission of stimuli. In disorders such as multiple sclerosis or leukodystrophy, the formation or function of the myelin is disturbed.
Previously, the molecular mechanisms of myelin formation were not well understood. Two projects undertaken by the Department of Molecular Cell Biology of the Faculty of Biology at the Johannes Gutenberg University in Mainz have now made a significant contribution towards understanding these complex cellular processes.
In simple terms, signals transmitted during saltatory conduction jump from one non-myelinated area (the nodes of Ranvier) to another, which enormously increases the speed of transmission. Myelin is formed in the central nervous system when oligodendrocytes - a specific type of brain cell - wrap their cellular extensions around the axons of the nerve cells several times, thus forming a compact stack of cellular membranes.
The team of scientists under Professor Jacqueline Trotter from the Mainz Department of Molecular Cell Biology have now been able to show which mechanisms contribute towards the formation of an intact myelin sheath and how the nerve cells control the place and time of myelin production.
Brain cells known as neurons process information by joining into complex networks, transmitting signals to each other across junctions called synapses. But "neurons don't just connect to other neurons," emphasizes Z. Josh Huang, Ph.D., "in a lot of cases, they connect to very specific partners, at particular spots." Dr. Huang, a professor at Cold Spring Harbor Laboratory (CSHL), leads a team that has identified molecules guiding this highly specific neuronal targeting in the developing brains of mice.
The researchers report in PLoS Biology that in some cases, these molecular guides -- non-signaling brain cells known as glia -- form a kind of scaffold. This scaffold, in turn, directs the growth of nerve fibers and their connections between specific types of neurons.
As they learn through research like this how the brain develops its complex wiring, the scientists hope they can clarify what goes wrong in disorders like autism.