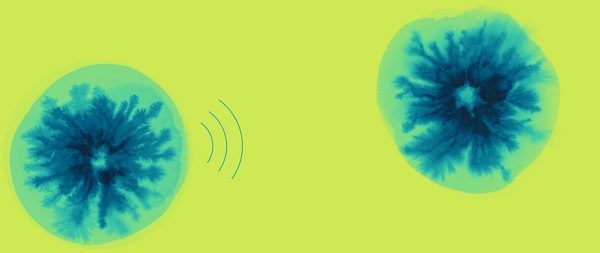
The preferred form of community for bacteria seems to be the biofilm. On teeth, on pipes, on rocks and in the ocean, microbes glom together by the billions and build sticky organic superstructures around themselves. In these films, bacteria can divide labor: Exterior cells may fend off threats, while interior cells produce food. And like humans, who have succeeded in large part by cooperating with each other, bacteria thrive in communities. Antibiotics that easily dispatch free-swimming cells often prove useless against the same types of cells when they've hunkered down in a film.
As in all communities, cohabiting bacteria need ways to exchange messages. Biologists have known for decades that bacteria can use chemical cues to coordinate their behavior. The best-known example, elucidated by Bonnie Bassler of Princeton University and others, is quorum sensing, a process by which bacteria extrude signaling molecules until a high enough concentration triggers cells to form a biofilm or initiate some other collective behavior.
But Süel and other scientists are now finding that bacteria in biofilms can also talk to one another electrically. Biofilms appear to use electrically charged particles to organize and synchronize activities across large expanses. This electrical exchange has proved so powerful that biofilms even use it to recruit new bacteria from their surroundings, and to negotiate with neighboring biofilms for their mutual well-being.
"I think these are arguably the most important developments in microbiology in the last couple years," said Ned Wingreen, a biophysicist who researches quorum sensing at Princeton. "We're learning about an entirely new mode of communication."
Biofilms were already a hot topic when Süel started focusing on them as a young professor recruited to San Diego in 2012. But much about them was still mysterious, including how individual bacteria give up their freedom and settle into large, stationary societies. To gain insight, Süel and his colleagues grew biofilms of Bacillus subtilis, a commonly studied rod-shaped bacterium, and observed them for hours with sophisticated microscopes. In time-lapse movies, they saw biofilms expand outward until cells in the interior consumed the available reserves of the amino acid glutamate, which the bacteria use as a nitrogen source. Then the biofilms would stop expanding until the glutamate was replenished. Süel and his colleagues became curious about how the inner bacteria were telling the outer cells when to divide and when to chill.
Quorum sensing was the obvious suspect. But Süel, who was trained in physics, suspected that something more than the diffusion of chemical messengers was at work in his Bacillus colonies. He focused on ion channels - specialized molecules that nestle into cells' outer membranes and ferry electrically charged particles in and out. Ion channels are probably most famous for their role in nerve cells, or neurons. Most of the time, neurons pump out sodium ions, which carry a single positive charge, and let in a different number of potassium ions, also with single positive charges. The resulting charge imbalance acts like water piling up behind a dam. When an electrical impulse jolts a neuron's membrane, specialized channels open to allow the concentrated ions to flood in and out, essentially opening the dam's floodgates. This exchange propagates along the neuron, creating the electrical "action potentials" that carry information in the brain.
Süel knew that bacteria also pump ions across their membranes, and several recent papers had reported spikes of electrical activity in bacteria that at least loosely resembled those found in the brain. Could bacteria also be using the action-potential mechanism to transmit electrical signals? he wondered.
He and his colleagues treated biofilms in their lab with fluorescent markers that are activated by potassium and sodium ions, and the potassium marker lit up as ions flowed out of starved cells. When the ions reached nearby cells, those cells also released potassium, refreshing the signal. The signal flowed outward in this way until it reached the biofilm's edge. And in response to the signal, edge cells stopped dividing until the interior cells could get a meal, after which they stopped releasing potassium.
Süel's team then created mutant bacteria without potassium channels, and they found that the cells did not grow in the same stop-start manner. (The researchers also saw no movement of labeled sodium ions in their experiments.) Like neurons, bacteria apparently use potassium ions to propagate electrical signals, Süel and his colleagues reported in Nature in 2015.
Reader Comments
to our Newsletter