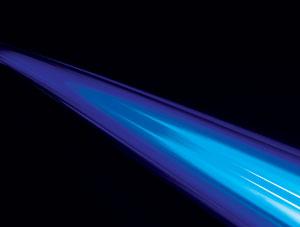
© John Rensten/Photodisc/GettyA new kind of laser could mean cheaper gadgets for all
Lasers might be pushing 50, but they are still the youthful pin-ups of fundamental physics. Since the first one was unveiled in 1960, the more apocalyptic predictions of how they might be used - as death rays, for example - have proved to be overblown. Their peaceful application, on the other hand, can be seen everywhere from cutting and welding to combating cancer and cataracts, to powering telecoms and consumer electronics, and has mushroomed into an industry worth $6 billion in 2007. Advances in the laser lab translate into gadgets in our homes at astonishing speed: think of the progression from CD to DVD and now Blu-ray technology in just a few decades.
So here's a heads-up for you: we could be about to witness the next stage in the laser's evolution, a sea change in how laser light is produced. A new wave of devices looks likely to exploit particle-like packets of energy to produce their light - packets that are neither light, nor matter, but both. It's early days, but advances in taming these exotic beasts are proceeding apace. "I keep expecting progress to end," says physicist Jeremy Baumberg at the University of Cambridge, one of the pioneers. "But it just keeps going." The pay-offs could be immense: not just lasers that use less juice than ever before and new low-power lighting technology but maybe even a way to make the semi-mythical, superpowerful quantum computer.
What is this new item in the physicist's armoury? The polariton, or exciton polariton, to give it its full name. How can such a particle be light and matter simultaneously? As is so often the case, the secret lies in the weirdness of quantum physics. Polaritons are not the kind of beasts you can expect to see in the wild. First bred in 1991 by researchers at the University of Tokyo, Japan, they spend the entirety of their short lives in tiny mirrored cages known as semiconductor microcavities.
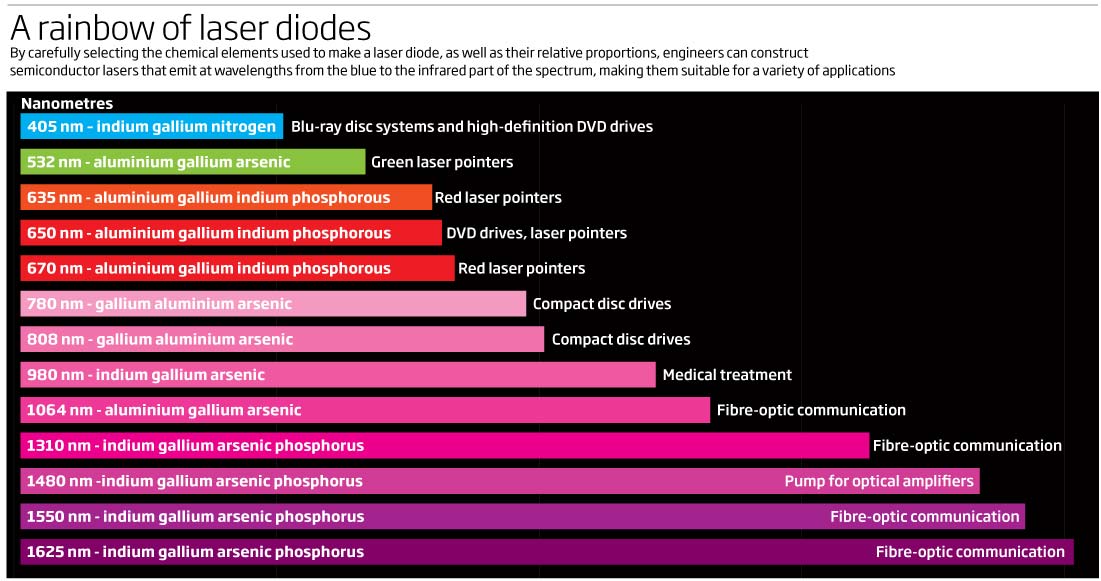
© UnknownA rainbow of laser diodes
The gestation of polaritons is a complex process. It begins in a sandwich of semiconducting materials known as a quantum well. Electrons are jammed tightly into the thin, sheet-like filling of this sandwich - typically less than a micrometre thick - and so are particularly excitable. Add a little drop of energy, in the form of light or a voltage, and some of the electrons absorb it and jump to a higher energy level, leaving behind an absence of electrons - positively-charged "holes". An electron-and-hole pairing is called an exciton, and is usually a short-lived affair: the energised electron soon gives up its extra energy and plonks itself back into the hole. At the same time it releases the energy it had taken on board, in the form of a photon of light.
This is where the cunning physics comes in. Place the quantum well between a pair of highly polished mirrors to create a cavity about 1 micrometre long, and now the photon will ping back into the system to create another exciton, which in turn emits its energy as another photon. By carefully controlling the distance between the mirrors, the photons or light waves being emitted by many excitons can be made to build in intensity and resonate with one another, like the vibrations of a vigorously bowed violin string. This creates yet more excitons which produce more resonant light waves and so on, until energy begins to cycle between light and matter so fast - in just a few trillionths of a second - that according to the rules of quantum physics it becomes impossible to tell in which of the two states it is stored. And that is a polariton. Matter in the form of electrons and photons come together to make it, but its precise identity remains unclear. "You really get into a twist if you try to think of polaritons as half matter and half light," says Baumberg. "They're something completely different."
And very odd characters they turn out to be. Take their mass, for example. Electrons are hardly the most heavyweight of particles, but compared with massless photons they are distinctly beefy. As a polariton contains a whole electron, you might expect its mass to be pretty close to the electron's. Not so. In fact, it seems to be only about one ten-thousandth the mass of the electron.
Or take the way polaritons behave in a group. While electrons repulse their own kind, declining to exist in the same quantum energy states, polaritons are quite the reverse, liking nothing better than to get up close and personal by adopting a common, or "coherent", quantum state. As a result, polaritons have proved themselves supremely adept at generating coherent laser light.
To a physicist, expecting polaritons to be able to generate laser light is an obvious leap of faith: the lasers in consumer electronic devices use similar materials and processes to those used to produce polaritons. Laser light is produced in a semiconductor quantum well by kicking electrons up into an energised state so that more of them are excited than not. The excited electrons then fall back down again, emitting photons of identical frequency and phase which can be focused into a coherent light beam.
This process is not helped by the electrons' stand-offishness, which means that no two electrons will share the same quantum state. So electrical engineers resort to brute force and pump in a substantial amount of energy - in the form of light or electric current - to force electrons upwards and thus kick-start the lasing process.
By comparison, lasing with polaritons may be a piece of cake. Just a small amount of energy should be capable of initiating a resonant transition between light and matter. The polaritons created are only too happy to crowd into the same quantum state, emitting coherent laser light when they decay.
Experiments have not disappointed. Baumberg led a group from the universities of Sheffield and Southampton, both in the UK, that in 2000 first demonstrated the promise of lasers using polaritons. By shining laser light into a quantum well at an angle carefully chosen to hit the confined electrons' sweet spot, they found they could amplify the light and that this amplification increased exponentially as the power of the input laser was increased, reaching a factor close to 100 (
Physical Review Letters, vol 84, p 1547). "It was a bigger light gain than in any known material," says Baumberg.
Despite this spectacular start, the race towards practical polariton lasers has since encountered a few hurdles. Gallium arsenide was an obvious choice of material for the first experiments because it forms the basis of one of the best-developed families of semiconductor lasers, whose infrared light is used in hundreds of millions of CD and DVD players (see chart). Unfortunately, it turned out to be anything but ideal as an environment in which to nurture polaritons. The initial research of Baumberg and colleagues was done at ultralow temperatures of between 10 and 50 kelvin, but as soon as the thermostat was raised to anything approaching room temperature, increased thermal jiggling caused the polaritons to break up. "They were simply too delicate to survive," says Pavlos Savvidis, who was a research student at the University of Southampton at the time.
So the search was on for an alternative semiconductor in which polaritons might feel more at home. It was soon found in a semiconductor based on gallium nitride, a material that has been used to make very bright blue laser diodes since the 1990s. The relatively small wavelength of blue light means it can etch information on a storage medium and then read it back again on much smaller scales than the infrared lasers used in CD and DVD technologies can. This is the secret behind the high-definition storage capabilities of Blu-ray discs.
In gallium nitride-based quantum wells, polaritons could be held stable even at room temperature. The problems were not solved yet, however. "Gallium nitride's structure is far more disordered than gallium arsenide's," explains Jacqueline Bloch, who leads a group developing polariton lasers at the Laboratory of Photonics and Nanostructures in Marcoussis near Paris, France. That disorder makes it far more difficult to get light rattling around in the mirrored cage in the resonant way needed to induce polariton lasing. It sparked a major Europe-wide initiative to design semiconductor cavities that could confine photons better.
This finally paid off in 2007, when researchers at the University of Southampton and at the Swiss Federal Polytechnic Institute in Lausanne (EPFL) announced that they had induced polariton lasing in gallium nitride at room temperature - at a threshold energy just one-tenth of that needed in a conventional laser (
Physical Review Letters, vol 98, p 126405). This could be the breakthrough that industry is waiting for, Baumberg reckons. "Blu-ray technology is expensive because the gallium nitride lasers in it often fail," he says. With a lower power threshold, polariton lasers promise better reliability, at a lower price.
Yet there is still a crucial hurdle to overcome before polariton lasers can break into the mainstream. All successful polariton lasing experiments so far have used light from another laser to kick-start the process. For consumer electronics, an electrical current is the preferred method. Working out how to build current-triggered polariton devices will be a fiddly business, says Bloch, as it will place even higher demands on the design of the microcavity. Nevertheless, her group and others tackling this challenge are optimistic of success within the next few years.
Batteries are betterSavvidis, now based in Greece at the University of Crete in Heraklion, has not given up on gallium arsenide in the meantime. He and his colleagues announced in May 2008 that they had produced an electrically driven polariton light-emitting diode (LED) in gallium arsenide that worked at a comparatively balmy temperature of 235 kelvin (-38 °C) (
Nature, vol 453, p 372). A demonstration at room temperature followed in February 2009 (
Applied Physics Letters, vol 94, p 071109). An LED is an intermediate step on the way to a laser, a low-intensity light source that does not demand the kind of coherent emission that lasers do. Once restricted to the standby indicators on televisions, LEDs are technologically important in their own right, however. As the intensities and range of colours available increase, they are rapidly spreading to all sorts of lighting applications as a super-efficient alternative to traditional incandescent bulbs.
The promise of polaritons does not end in power-saving light sources. According to physicist Leonid Butov at the University of California, San Diego, they could be ideal candidates to take the place of electron currents in a future generation of super-efficient computers. Conventional computers are adept at processing information but not so good at moving it about, he says. The pliability of electrons comes in handy when manipulating data on a silicon chip, but transporting this data increasingly exploits the zippiness of photons in optical fibres, rather than electrons in copper wires.
Switching the information between electron and photons and back again is a messy and time-consuming business - not helped by the fact that it is difficult to make silicon emit light. Here, the hybrid nature of polaritons could come to the rescue. "Because you have a real particle in there with mass, you can apply logic gates and actually control polaritons," says Butov. It would then be relatively straightforward to extract the light from the polaritons and use that to transport the stored information. In July 2008, Butov and colleagues published details of a transistor - the basic switching element of a logic circuit - that uses excitons, the matter precursors of polaritons (
Science, vol 321, p 229). That same month, a theory paper detailed how something similar might be achieved with polaritons (
Physical Review Letters, vol 101, p 016402). In December 2008, Bloch's group reported the first demonstration of polariton switching in a gallium arsenide diode cooled to 10 kelvin (
Physical Review Letters, vol 101, p 266402). A similar device could form the building block of a polariton transistor.
In his lab at EPFL, Benoît Deveaud-Plédran is looking even further ahead. He thinks polaritons might point the way towards a workable quantum computer. Such a computer would work by manipulating not currents of particles, but the properties of the particles themselves. In particular, they would use quantum sorcery to entangle the states of the particles and transport information between particles.
One scheme for creating these entangled particles involves the use of an exotic state of matter called a Bose-Einstein condensate. Neither solid, liquid or gas, such a collection of atoms behaves in the same weird quantum ways as a single atom but on a larger scale, making those properties much easier to observe. Unfortunately, such atomic Bose-Einstein condensates only form within a fraction of a degree of absolute zero, which makes probing their properties a tough ask, never mind harnessing them as a computer.
In 2006, however, a team of British, French and Swiss researchers announced something that mimicked the behaviour of a Bose-Einstein condensate in a semiconductor cavity containing polaritons cooled to a slightly less frigid 19 kelvin (
Nature, vol 443, p 409). Crucially, calculations showed that because polaritons are much lighter than atoms, such condensation should also be possible at much higher temperatures - even at room temperature. "Bose-Einstein condensation is an extraordinary phenomenon with all sorts of crazy properties, such as superfluidity," says Deveaud-Plédran, who was one of the team. "Doing that at room temperature would be something special."
Deveaud-Plédran suspects that polariton condensates could offer some other useful advantages. With polaritons, you could produce entangled states in a solid material such as a semiconductor, manipulate the states - do computing - and then break them up and have the results of the calculation encoded in the light, he suggests. At the very least, Bloch says, polaritons should eventually provide a room-temperature source of identical photons - an essential resource for anyone developing encoding schemes for use in quantum cryptography.
Such suggestions are for the future, of course. Having witnessed how fast things have developed so far, no one is excluding any possibility. Baumberg certainly isn't. "The polariton laser was the first thing I started working on as an academic, and it is the one thing I didn't patent," he says. "Now I'm thinking I should have done."
Reader Comments
to our Newsletter