MAGIC - the Major Atmospheric Gamma-ray Imaging Cherenkov Telescope - scans the sky each night for high-energy photons from the distant cosmos. Most nights, nothing remarkable comes. But every now and again, a brief flash of energetic light bears witness to the violent convulsions of a faraway galaxy.
What MAGIC saw on that balmy June night came like a bolt from the blue. That is because something truly astounding may have been encoded in that fleeting Atlantic glow: evidence that the fabric of space-time is not silky smooth as Einstein and many others have presumed, but rough, turbulent and fundamentally grainy stuff.
It is an audacious claim that, if verified, would put us squarely on the road to a quantum theory of gravity and on towards the long-elusive "theory of everything". If it were based on a single chunk of MAGIC data, it might easily be dismissed as a midsummer night's dream. But it is not. Since that first sighting, other telescopes have started to see similar patterns. Is this a physics revolution through the barrel of a telescope?
Such incendiary thoughts were far away from Robert Wagner's mind when the MAGIC data filtered through to the Max Planck Institute of Physics in Munich, Germany, the morning after. He and his fellow collaborators were enjoying a barbecue. Not for long. "We put our beers aside and started downloading the full data set," says Wagner.
It was easy to pinpoint the source of the data blip - a 20-minute burst of hugely energetic gamma rays from a galaxy some 500 million light years away known as Markarian 501. Its occasional tempestuous outbursts had already made it familiar to gamma-ray telescopes worldwide.
This burst was different. As Wagner and his colleagues analysed the data in the weeks and months that followed, an odd pattern emerged. Lower-energy photons from Markarian 501 had outpaced their higher-energy counterparts, arriving up to 4 minutes earlier (Physics Letters B, vol 668, p 253).
This should not happen. If an object is 500 million light years away, light from it always takes 500 million years to get to us, no more, no less. Whatever their energy, photons always travel at the same speed, the implacable cosmic speed limit: the speed of light.
Perhaps the anomaly has a mundane explanation. We do not really understand the processes within objects such as Markarian 501 that accelerate particles to phenomenal energies and catapult them towards us. They are thought ultimately to have something to do with the convulsions of supermassive black holes at the objects' hearts. It could be that these mechanisms naturally spew out low-energy particles before high-energy ones.
Or they might not. "The more fascinating explanation would be that this delay is not intrinsic to the source, but that it happens along the way from the source to us," says Wagner.
Quantum signature
What piqued the interest of Wagner and his colleagues was that the MAGIC observations were showing just the sort of effect that quite a few models of quantum gravity predict. Physicists have been on the lookout for experimental signposts to the right theory for the best part of a century (see Quantum gravity: why we care).
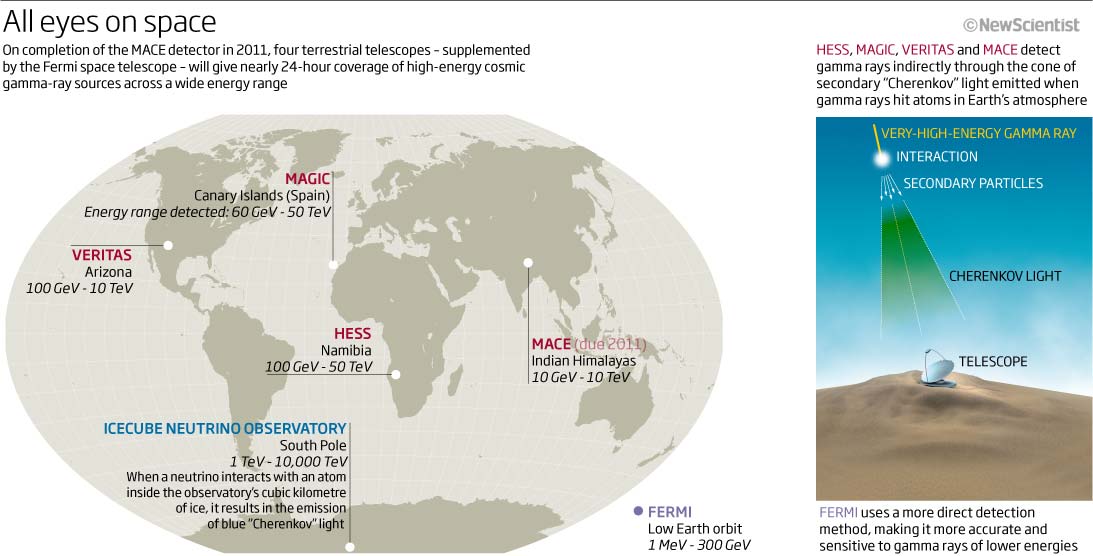
Last year, it was suggested that the signature of just such a quantum space-time had popped up in unexplained noise plaguing a gravitational-wave detector in northern Germany (New Scientist, 17 January 2009, p 24). But that interpretation is far from a done deal, and most experts agree that a more substantive sighting could only come from observing the possible interactions of space-time with particles passing through it.
According to many string theory models, particles of different energies should speed up or slow down by different amounts as they interact with a foamy space-time. A minimum size for space-time grains, as predicted by loop quantum gravity, could violate the cherished principle of special relativity known as Lorentz invariance, which states that the maximum speed of all particles, regardless of their energy, is the speed of light in a vacuum.
The trouble is that these effects would be observable only with particles far more energetic than even the beefiest terrestrial particle accelerators can produce. Even if we could make these particles, the tiny interactions between them and the fabric of space-time would not add up to a hill of beans, even over many laps of the Large Hadron Collider's 27-kilometre-long loop at CERN, near Geneva, Switzerland.
Summed over hundreds of millions or billions of light years, such interactions could account for the MAGIC travel-time anomaly. It looks like nature might have provided us with particle accelerators - distant galaxies - whose products could, for the first time, allow us to test predictions of quantum gravity against hard experimental evidence.
As yet, we have only seen a handful of gamma-ray bursts of the energy and intensity needed to see whether the delay effect is a consistent feature. In July 2006, the High Energy Stereoscopic System (HESS), an array of gamma-ray telescopes in the desert of Namibia, saw a high-energy flare erupt from an active galaxy nearly four times as far away as Markarian 501. The burst contained marginal evidence for a time-lag of around half a minute for the most energetic photons, which were considerably less energetic than those in the flare spotted by MAGIC. The uncertainties in the data resulting from the detection process, however, made a definitive statement impossible (Physical Review Letters, vol 101, p 170402).
It is recent results from NASA's Fermi Gamma-ray Space Telescope, launched last year, that provide the most tantalising glimpse yet of something extraordinary going on out there. Last September, it spied a burst of gamma rays from a source nearly 12 billion light years away. According to an analysis by Amelino-Camelia and Lee Smolin of the Perimeter Institute for Theoretical Physics in Waterloo, Canada, the zippiest low-energy photons beat some of the high-energy stragglers to Earth by anything up to 20 minutes. Two much closer bursts seem to contain much smaller delays.
The individual observations are pretty consistent with each other, too, says theorist John Ellis at CERN. He and colleagues have taken data from the MAGIC and HESS bursts to calibrate a theoretical model inspired by string theory that assumes the delay effect increases linearly with distance and photon energy. Using it to estimate the delay that the highest-energy photon in the Fermi space telescope's September burst should have experienced, they came up with a figure of 25 seconds, plus or minus 11 seconds. What Fermi had measured for that particular photon was 16.5 seconds - within the model prediction's admittedly large margin of error.
The only way to find out conclusively whether the delays are a consistent signature of a quantised or foam-like space-time, says Ellis, is to get more data - ideally from sources at many different distances. "Then we'll be able to see whether we can distinguish between effects at the source and effects in the propagation," he says.
Worldwide cover
We also need to observe the same burst with more than one instrument. Each telescope is sensitive to a different energy range, owing to its altitude and detector set-up. Combining different data sets will provide a wider spread of energies from which to tease out any energy-dependent effect, and also help us get round a persistent irritant to consistent astronomical observations: Earth's rotation. Not only does our planet's spin mean that multitudes of photons from the sun overwhelm any cosmic source for a large proportion of the day, but it also makes observing a highly directed beam of gamma rays from one specific direction tricky, even at night: as you train your telescope on your target, the Earth moves beneath your feet and eventually the source slips out of sight.
That means MAGIC can observe any burst for a maximum of only 6 hours on any given night, assuming it is pointing in the right direction when a new burst arrives. That period could be doubled by using it in conjunction with a similar instrument - the Very Energetic Radiation Imaging Telescope Array System (VERITAS) - that sits atop Mount Hopkins in southern Arizona.
A further gamma-ray telescope, the Major Atmospheric Cherenkov Telescope Experiment (MACE), 4500 metres up on the Tibetan plateau in the remote region of Ladakh, India, will open that observational window still further. When completed in 2011, MACE will be the highest-altitude gamma-ray telescope in the world, capable of observing gamma rays with a wide range of energies. "Then we will have another observatory 5 to 6 hours in front of MAGIC," says Wagner. "That could lead the way to a continuous, 24-hour observation of certain objects."
What with that and the new high-accuracy data from the Fermi space telescope, gamma ray telescopes could well uncover quantum space-time within the next few years. Even so, they still might be beaten to the line. The definitive answer might come from a very different source, and a very different quarter of Earth's surface - the South Pole.
That is because a cubic kilometre of ice under the South Pole will soon be home to the IceCube Neutrino Observatory, whose strings of detectors will watch for faint flashes of blue light emitted when neutrinos from cosmic sources smash into the Antarctic ice.
Neutrinos are ghostly particles thought to be produced in the same violent events that produce high-energy gamma rays. As yet, we have not seen any neutrinos from outside our galaxy, barring some that burst on us from a supernova in a neighbouring galaxy, the Large Magellanic Cloud, in 1987. The neutrinos we do see are lower-energy ones that come from nuclear reactions in the sun and particle interactions in Earth's atmosphere. IceCube aims to change that.
And it could see something big. Because the quantum-mechanical wavelengths associated with neutrinos of the very highest energies are even smaller than those of high-energy photons, they could be more susceptible to disruption through interactions with a space-time that is grainy on very small scales. Francis Halzen of the University of Wisconsin, Madison, who leads the IceCube experiment, has calculated together with his colleagues that in one favoured model of quantum space-time such interactions could dramatically speed up higher-energy neutrinos (Physical Review D, vol 72, p 065019). "It's a beautiful signal that could not be explained by conventional astrophysics," he says.
Humble constructions
That's not the only attractive property of neutrinos when it comes to testing the idea of a frothy space-time, says Dan Hooper of Fermilab in Batavia, Illinois. Neutrinos come in three distinct "flavours", named after the chunkier particles they are associated with - the electron, the muon and the tau. They tend to morph back and forth between these different states as they travel, a phenomenon known as neutrino oscillation. If a distant source is emitting only electron neutrinos, theory tells us how many should have changed flavours by the time they reach us.
If neutrinos were interacting with the quantum foam, though, they would forget their original flavour along the way, leading to equal numbers of all flavours by the time they arrive here. "That effect would be hard to explain with normal astrophysics," says Hooper. He suggests a possible, albeit disputed, source of electron neutrinos in the Cygnus region of the Milky Way that could be ripe for investigation (Physics Letters B, vol 609, p 206).
Uncertainties in models of neutrino oscillations make exact calculations of the expected extent of the flavour-equalising effect difficult, as Hooper himself points out. And even if we do strike it lucky and find indisputable signs that either neutrinos or gamma rays are being affected by the structure of space-time, it will be a long, hard slog to convert that evidence into a viable theory of quantum gravity. Amelino-Camelia likens the situation now to that of a century ago, when anomalous observations - such as the spectrum of black-body radiation, or the photoelectric effect - that could not be explained by classical means set physics on the decades-long path towards a fully fledged quantum theory. It did not come easy.
And so it will be for quantum gravity. "We have to build, humbly, very humbly, from what we know," says Amelino-Camelia. "Construct simple theories, which are very far from being a theory of everything, but intelligible enough that they can guide us to the next spark." Whether on Atlantic islands, in the Himalayas, deep in the Antarctic ice or high above Earth's atmosphere, watchful eyes are waiting for signs from the universe's quantum fabric.
Gallery: All eyes on space: The global gamma-ray network
Just be prepared to repeat everything you were told. That is necessary to prove your fitness as a scientist.
The emperor's new clothes are very beautiful, are they not?